the Creative Commons Attribution 4.0 License.
the Creative Commons Attribution 4.0 License.
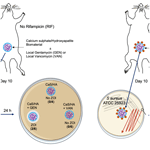
Systemic rifampicin shows accretion to locally implanted hydroxyapatite particles in a rat abdominal muscle pouch model
Sujeesh Sebastian
Jintian Huang
Yang Liu
Mattias Collin
Magnus Tägil
Deepak Bushan Raina
Lars Lidgren
Introduction: biomaterials combined with antibiotics are routinely used for the management of bone infections. After eluting high concentrations of antibiotics during the first week, sub-inhibitory concentrations of antibiotics may lead to late repopulation of recalcitrant bacteria. Recent studies have shown that systemically given antibiotics like tetracycline and rifampicin (RIF) could seek and bind to locally implanted hydroxyapatite (HA). The aim of this in vivo study was to test if systemically administered rifampicin could replenish HA-based biomaterials with or without prior antibiotic loading to protect the material from late bacterial repopulation. Methods: in vivo accretion of systemically administered RIF to three different types of HA-based materials was tested. In group 1, nano (n)- and micro (m)-sized HA particles were used, while group 2 consisted of a calcium sulfatehydroxyapatite (CaSHA) biomaterial without preloaded antibiotics gentamycin (GEN) or vancomycin (VAN), and in group 3, the CaSHA material contained GEN (CaSHA+GEN) or VAN (CaSHA+VAN). The above materials were implanted in an abdominal muscle pouch model in rats, and at 7 d post-surgery, the animals were assigned to a control group (i.e., no systemic antibiotic) and a test group (i.e., animals receiving one single intraperitoneal injection of RIF each day (4 mg per rat) for 3 consecutive days). Twenty-four hours after the third injection, the animals were sacrificed and the implanted pellets were retrieved and tested against Staphylococcus aureus ATCC 25923 in an agar diffusion assay. After overnight incubation, the zone of inhibition (ZOI) around the pellets were measured. Results: in the control group, CaSHA+GEN pellets had a ZOI, while all other harvested pellets had no ZOI. No pellets from animals in test group 1 had a ZOI. In test group 2, CaSHA pellets showed a ZOI. In test group 3, CaSHA+GEN and CaSHA+VAN pellets showed a ZOI. Conclusions: in this proof-of-concept study, we have shown that a locally implanted biphasic CaSHA carrier after 1 week can be loaded by systemic RIF administration and exert an antibacterial effect. Further in vivo infection models are necessary to validate our findings.
- Article
(8130 KB) - Full-text XML
- BibTeX
- EndNote
In bone infections, targeted antibiotic delivery using a carrier has become an established treatment, as it gives high local concentrations and manages dead space with less systemic side effects (Hanssen and Spangehl, 2004; Henry and Galloway, 1995). Following the introduction of antibiotic-containing polymethylmethacrylate (PMMA) bone cement, various resorbable and non-resorbable carriers were developed (Buchholz and Engelbrecht, 1970; Kanellakopoulou and Giamarellos-Bourboulis, 2000; McNally et al., 2016). Among the resorbable antibiotic carriers, biphasic calcium sulfate/hydroxyapatite (CaSHA) bone void filler has shown encouraging clinical outcomes in the treatment of bone infections (McNally et al., 2016, 2022; Stravinskas et al., 2018). Antibiotic release kinetics is highly dependent on the chemical interactions between the antibiotic and the components of the carrier material (Funk et al., 2021; Stravinskas et al., 2016; Stravinskas et al., 2019). After eluting high effective concentrations of antibiotics initially, non-degradable carriers release sub-inhibitory levels of antibiotics, which may lead to resistance development and infection recurrence (Anagnostakos et al., 2008, 2009; Gimza and Cassat, 2021; Neut et al., 2001; Sebastian et al., 2019). Especially PMMA was reported to become a prime surface for bacterial biofilm formation and infection recurrence (Neut et al., 2001; Sebastian et al., 2019). Currently, there is no method available for the clinicians to reload these carriers with antibiotics to treat lingering infections and to prevent bacterial attachment.
Using the unique binding properties of hydroxyapatite (HA), a major component in bone, recently Raina et al. (2020a) showed the concept of biomodulation, a novel method of targeted drug delivery, in a rat abdominal muscle pouch model. They demonstrated that a locally implanted particulate HA-containing biomaterial could act as a recruiting moiety for systemically administered HA-seeking drugs, including the antibiotic tetracycline (TET) (Raina et al., 2020a). In addition, using zoledronic acid, the authors showcased the possibility of drug reloading of a CaSHA biomaterial. In a subsequent study, Sebastian et al. (2022a) demonstrated the antibacterial effect of locally implanted HA particles biomodulated with TET. Antibiotics other than TET like rifampicin (RIF) and daptomycin are capable of accretion to HA, potentially by chemically interacting with the calcium, phosphate, or hydroxyl groups on the HA crystal (Raina et al., 2020b). However, none of these previous studies reported systemic loading of a locally implanted carrier already containing an antibiotic.
RIF is a valuable part of the armamentarium in the treatment of osteomyelitis, prosthetic joint infections, and tuberculosis (Osmon et al., 2013). Bone infections caused by Staphylococcus aureus often include a minimum of 2–6 weeks treatment with oral RIF combined with an additional antibiotic given either intravenously or orally (Chen et al., 2022; Depypere et al., 2020; Osmon et al., 2013; Sebastian et al., 2021; Sendi and Zimmerli, 2017; Zimmerli and Sendi, 2019). A recent in vitro study showed that staphylococcal bone infection significantly impairs metabolic and bone formation activities of osteoblasts, and treatment with RIF may enhance bone regeneration (Alagboso et al., 2022). However, diffusion of systemically administered antibiotics into sclerotic bone is difficult (Chen et al., 2022). We recently published an in vitro study on the local addition of RIF to CaSHA containing either gentamicin (GEN) or vancomycin (VAN) and verified extended antibacterial and biofilm effect with no viable bacteria (S. aureus) until study endpoint (35 d) (Sebastian et al., 2022b). The strains developed resistance when only RIF was added but not when combined with GEN or VAN (Sebastian et al., 2022b). However, no clinically approved RIF-containing carriers are today available for local delivery. In addition, there is a need for new techniques to improve the local concentration of cornerstone antibiotic for bone infections like RIF. Globally, there is an alarming increase in antibiotic resistance, and all antibiotic treatment modalities should be guided by an active antibiotic stewardship.
The primary aim of this study was therefore to evaluate a novel method to load and protect an antibiotic carrier material from bacterial re-population by systemically administering RIF. In a non-infectious in vivo setting, pellets of commercially available and clinically used CaSHA ceramic carrier containing GEN or VAN (Cerament® G or Cerament® V, Bonesupport AB, Sweden) were implanted in rat abdominal muscle pouches, and 7 d post operatively, systemic administration of RIF at clinical doses was given. The antibacterial effect of the antibiotic reloaded pellets was evaluated in vitro on planktonic bacteria. As a secondary objective, using the same methods, we also tested the RIF-seeking ability of synthetic, medical grade nano-/micro-sized HA particles (Fluidnova, S.A., Portugal) and clinically used CaSHA biomaterial (Cerament® bone void filler, Bonesupport AB, Sweden) without any prior added antibiotic.
2.1 Materials used
Nano (n)- and micro (m)-HA powder of < 50 nm and 10 µm particle size, respectively, were purchased from Fluidnova, S.A., Portugal. Medical grade rifampicin (Rifampicin Ebb, 600 mg; Sanofi S.p.A, Anagni, Italy) was purchased from the local pharmacy (Apoteket AB, Sweden). CaSHA composites without any antibiotics (Cerament® bone void filler) or containing GEN (Cerament® G) or VAN (Cerament® V) were purchased from Bonesupport AB, Lund, Sweden.
2.2 In vivo biomodulation
2.2.1 In vivo biomodulation of synthetic HA particles (group 1)
Pellets of n-/m-HA were prepared by mixing 100 mg of n-/m-HA with 60 and 80 µL of hyaluronic acid (HAD, 1 mg mL−1), respectively. Using the mixture, pellets were casted by transferring them to an elastic mold (Ø = 4.7 mm). Following an established muscle pouch model, eight male Sprague–Dawley (SD) rats (8–12 weeks old, average weight 363.5 g) were operated in the abdominal muscle, and each rat received n-HA (n=8) and m-HA (n=8) pellets on the right and left side of the abdominal midline, respectively (Fig. 1). At 7 d post-surgery, six animals received a single intraperitoneal injection of RIF each day (4 mg per rat) for 3 continuous days, and this group of animals formed the test group (n=6), while the remaining animals were used as control animals (n=2) and did not receive any RIF injection. Twenty-four hours after the third injection, the animals were sacrificed.
Implanted pellets devoid of muscle tissue were retrieved from the animals and placed in a 2 mL microcentrifuge tube. Similarly, bone (trabecular and cortical), kidney, and liver tissues were collected from three animals and muscle tissue from all animals. All samples were placed in liquid nitrogen and immediately crushed to form a slurry. Using agar diffusion assay, antibacterial effect of the slurry was tested by placing them on the Muller Hinton agar plates inoculated with bacterial suspension of Staphylococcus aureus ATCC 25923 (OD600=0.1) which is sensitive to Gen, VAN, and RIF tested in this study. After overnight incubation at 37 ∘C, the diameter of the zone of inhibition (ZOI) around the paste was measured.
2.2.2 In vivo biomodulation of ceramic bone void fillers (group 2)
Pellets of CaSHA were prepared by hand mixing 500 mg of CaSHA powder in a 48-well plate by adding 215 µL iodine-based mixing solution (Iohexol®, Bonesupport AB, Sweden), using a sterile wooden spatula for 30 s. Using the mixture, pellets were casted by transferring them to an elastic mold (Ø = 4.7 mm). Following the same surgical protocol mentioned previously, seven male SD rats (8–12 weeks old, average weight 484 g) were operated, and each rat received CaSHA pellets on the right and left side of the abdominal midline, respectively. At 7 d post-surgery, five animals received a single intraperitoneal injection of RIF each day (4 mg per rat) for 3 continuous days (test group, n=5), and remaining two animals did not receive any RIF injection (control group, n=2). All animals were sacrificed 24 h after the third injection and implanted pellets devoid of muscle tissue were retrieved. Similarly, bone (trabecular and cortical), kidney, and liver tissues were collected from three animals and muscle tissue from all animals. All samples were placed in liquid nitrogen, immediately crushed to form a slurry, and then used for agar diffusion assay.
2.2.3 In vivo biomodulation of antibiotic eluting ceramic carries (group 3)
Pellets of CaSHA containing antibiotics were prepared by hand mixing 500 mg of CaSHA powder (Cerament G/V, Bonesupport AB, Sweden) in a 48-well plate with GEN (10.35 mg) dissolved in 283.5 µL saline (Bonesupport AB, Sweden) or VAN (24.57 mg) dissolved in 215 µL iodine-based mixing solution, using a sterile wooden spatula for 30 s. Using the mixture, pellets were casted as mentioned previously. Twelve male SD rats (8–12 weeks old, average weight 364 g) were operated, and each rat received CaSHA-G (n=12, approximately each pellet contains 2.6 mg of GEN) and CaSHA-V (n=12, approximately each pellet contains 8.2 mg of VAN) pellets on the right and left side of the abdominal midline, respectively. At 7 d post-surgery, six animals received a single intraperitoneal injection of RIF each day (4 mg per rat) for 3 continuous days (test group, n=6), and remaining six animals did not receive RIF injection (control group, n=6). All animals were sacrificed 24 h after the third injection and implanted pellets devoid of muscle tissue were retrieved. Similarly, bone (trabecular and cortical), kidney, and liver tissues were collected from three animals and muscle tissue from all animals. All samples were placed in liquid nitrogen and immediately crushed to form a slurry and used for the agar diffusion assay.
2.3 Statistical analysis
All data were presented as mean ± standard deviation (SD). Details of statistical analysis are indicated in each figure legend. All data processing was carried out on GraphPad Prism version 9.1.2 for MacOS (GraphPad Software, San Diego, CA, USA).
3.1 In vivo biomodulation of synthetic HA particles (group 1)
The large ZOI (23.3 mm ± 2.1) shown by liver samples indicates the route of excretion of RIF and validity of the experimental model (Fig. 2b). No ZOI was observed with n-HA samples (Fig. 2a) and other tested tissue (Fig. 2b). Of the six m-HA samples, one showed a narrow ZOI (10 mm) (Fig. 2a). Overall, systemic RIF administration failed to biomodulate the tested synthetic HA particles (Fig. 2c).
3.2 In vivo biomodulation of ceramic bone void fillers (group 2)
None of the CaSHA pellets () from two control animals without RIF administration showed a ZOI (Appendix A1). No muscle tissue samples showed ZOI, but, as expected, large ZOI was observed in the liver samples (Fig. 3a and b). Of the 10 CaSHA pellets implanted in five animals (two pellets per animal), all of them showed ZOI (13.3 mm ± 1.6), confirming the recruitment of systemically administered RIF (Fig. 3a and b).
3.3 In vivo biomodulation of antibiotic eluting ceramic carries (group 3)
Both muscle and bone samples from animals with and without RIF injection showed no ZOI. Kidney samples from all animals showed ZOI indicating the route of excretion of GEN and VAN, whereas liver samples from animals that received systemic RIF injection only exhibited ZOI. Among the six animals without any systemic RIF injection, two CaSHA-GEN samples showed ZOI (5.2 mm ± 8), but none of the CaSHA-VAN showed any antibacterial effects (Fig. 4a–c). Of the animals that received systemic RIF, (9 mm ± 4.7) and (5.5 mm ± 4.3) CaSHA-GEN and CaSHA-VAN samples showed ZOI, respectively (Fig. 4a–c). Systemic RIF injection significantly improved the antibacterial effect of CaSHA-VAN. More CaSHA-GEN samples showed antibacterial effect following systemic RIF injection, but the difference was not significant.
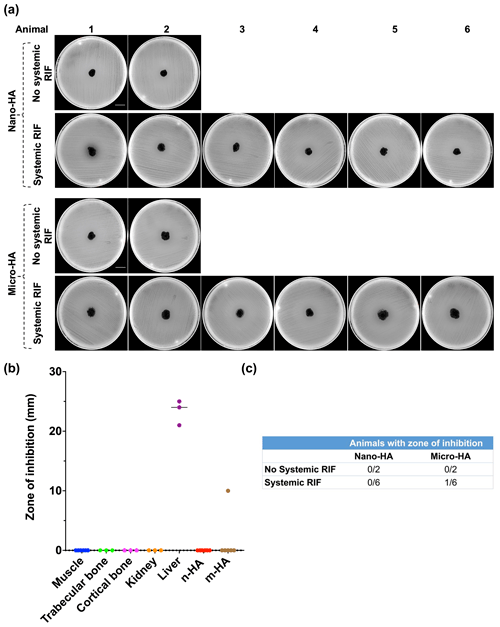
Figure 2In vivo antibiotic biomodulation of synthetic n-/m-hydroxyapatite particles after systemic rifampicin administration. (a) Muller Hinton agar plates inoculated with S. aureus ATCC 25923 showing no zone of inhibition (ZOI) of both n- and m-HA particles, except one m-HA sample. Scale bar is 2.1 cm. (b) Graph showing the ZOI of tissue samples and HA particles harvested from the test animals that received systemic RIF. HA and muscle samples were collected from animals, but bone, kidney and liver samples were collected only from . (c) Table showing the number of animals in the control and test groups wherein the implanted pellets of micro/nano HA exhibited a ZOI. Note: n-HA: nano-hydroxyapatite; m-HA: micro-hydroxyapatite.
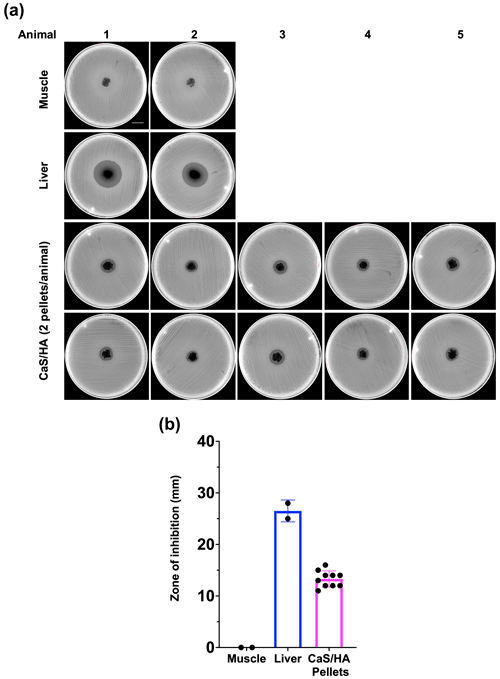
Figure 3In vivo antibiotic biomodulation of ceramic bone void filler CaSHA by systemic RIF administration. (a) Muller Hinton agar plates inoculated with S. aureus ATCC 25923 showing zone of inhibition (ZOI) by all RIF biomodulated CaSHA samples. All HA samples from n=5 animals (n=10 pellets) were collected from rats in the test group. And n=2 muscle samples and n=2 liver samples were also collected from the test group rats as controls. Scale bar is 2.1 cm. (b) Graph showing the ZOI of tissue and CaSHA samples harvested following RIF biomodulation.
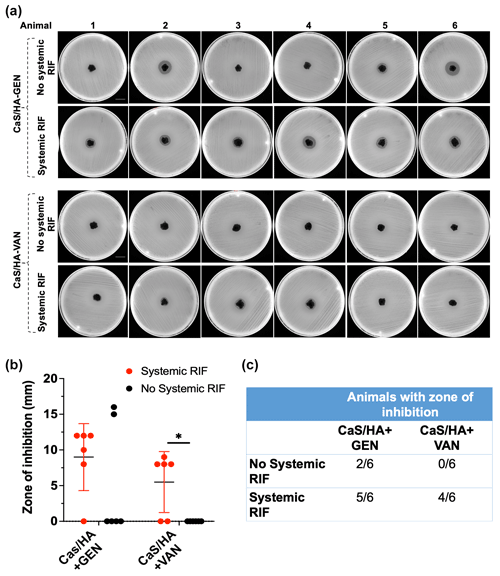
Figure 4Differences in the in vivo antibiotic biomodulation of gentamicin (GEN) or vancomycin (VAN) eluting CaSHA carriers (Cerament G/V) by systemic rifampicin (RIF) administration. (a) Agar diffusion assay showing the zone of inhibitions (ZOIs) exhibited by CaSHA-GENVAN pellets from animals with or without RIF injections. Scale bar is 2.1 cm. A total of n=6 CaSHA+GEN and CaSHA+VAN pellets were tested in the control group. Similarly, n=6 pellets of CaSHA+GEN and CaSHA+VAN were tested in the experiment group. (b, c) Graph and table showing the difference in the ZOI exhibited by CaSHA-GENVAN pellets with or without RIF injection. An unpaired t-test was used to compare the differences in ZOI. Note: * indicates p<0.05.
Systemic loading of locally implanted HA-based carriers using an HA-seeking antibiotic is an attractive option to improve the treatment efficacy in bone infections (Chen et al., 2022; Raina et al., 2020a, b). In this study, we found that CaSHA-based carriers could recruit an HA-seeking antibiotic like RIF to locally exert an adequate antibacterial effect at the targeted tissue. Considering the growing clinical use of biphasic ceramic carriers, our results provide new insights into obtaining extended effective local antibacterial levels beyond the first weeks. In addition, current findings open up a possibility of giving postoperative short-term systemic RIF to load a locally implanted antibiotic containing HA biomaterial, thereby increasing the efficacy. A recently published in vivo study reports that short-term administration of high dose RIF (human equipotent to 35 mg kg−1 d−1) ameliorated antimicrobial resistance in Staphylococcus aureus (Gordon et al., 2021). These results supplement our findings, and a higher but short-term postoperative RIF dosing regimen may further increase HA accretion and bacterial killing and thereby reduce the risk of developing resistance.
Accretion to n-and m-HA particles implanted in abdominal muscle pouches of rats failed except in one case with m-HA. In a landmark study by Raina et al. (2020a), CaSHA biomaterial containing m-HA particles in an ectopic implantation model recruited subcutaneously administered TET, an antibiotic known for its strong binding to HA particles, 2 weeks post-pellet implantation. By using a similar animal model, Liu et al. (2021) showed the recruitment of a systemically given anticancer drug, doxorubicin, by locally implanted n- and m-HA particles. In a recent study, using an abdominal subcutaneous pouch model, Qayoom et al. (2020) reported RIF accumulation in locally implanted biphasic n-HACaS ceramic carrier following continuous oral RIF administration (12 mg/animal) for 4 weeks. Contrary to their report, none of our n-HA samples showed any signs of RIF accretion. However due to differences in the type of n-HA, amount, and duration of RIF administered, a direct comparison of the two studies is not possible (Qayoom et al., 2020; Raina et al., 2020a). In addition, it has been reported that the method for HA synthesis could significantly affect the chemical and structural properties (Hossain et al., 2021; Vandiver et al., 2005).
Several studies have reported successful outcomes of biphasic injectable ceramic bone substitutes for bone remodeling in clinical conditions like fractures or benign bone tumors (Iundusi et al., 2015; Kaczmarczyk et al., 2015; Kotrych et al., 2018). Although resorption of CaS occurs at a fast phase during initial weeks, the HA particles have a slower resorption rate and they integrate with newly formed bone in a timespan of 6–12 months (Nilsson et al., 2013). This is important since we observed that HA particles can recruit systemically-given RIF and have an antibacterial effect. It has to be noted that contaminated open fractures always carry a high risk of getting a deep infection, especially using internal fixation devices (Metsemakers et al., 2018). One treatment approach would be to use the present standard, giving a conventional short-term antibiotic started early. In case a local antibiotic containing apatite is implanted in the fracture void, by giving additional systemic RIF, an antibacterial effective concentration could be achieved locally due to HA accretion. Although RIF is a recommended second line treatment option in bone infections, due to risk of resistance, its potential “protecting the protector” role needs further research, and its HA biomodulation capability should be tested when given as combination therapy.
In a prospective observational study, patients with trochanteric hip fractures who underwent internal fixation using dynamic hip screws and application of CaSHA-VAN, Stravinskas et al. (2019) measured VAN in urine for 20 d, indicating the elution of VAN from locally implanted CaSHA. Recently, an in vitro VAN release assay from CaSHA-VAN pellets showed elution of VAN levels above the minimum inhibitory concentration of S. aureus for 14–21 d (Sebastian et al., 2022b). Contrary to these reports, in the present study, CaSHA-VAN pellets did not show any antibacterial effects after day 10. However, because of the differences in the metabolic rates, physiological process, and pharmacokinetics, it has been reported that small lab animals do eliminate various antibiotics faster than humans (Freeman et al., 1997; Nair and Jacob, 2016). Since our study showed a significant antibacterial effect of CaSHA-VAN pellets following RIF biomodulation, clinically the ideal time of RIF loading could be after 7 d, but needs to be elucidated in further studies. Among six CaSHA-GEN pellets obtained from animals without any systemic RIF injection, two of them showed antibacterial effects. This indicates that in spite of a faster elimination of aminoglycoside antibiotics like GEN in rats, the locally implanted CaSHA still contains adequate levels of GEN (Freeman et al., 1997). Previous experimental and clinical studies also reported similar longer elution of GEN from CaSHA biomaterial (Sebastian et al., 2022b; Stravinskas et al., 2016). Considering this prolonged GEN elution from CaSHA, it could be argued that the ZOI shown by four of the six pellets from systemic RIF injection group may be from antibacterial effects conferred by remaining GEN in the pellets rather than accretion of RIF. Since more animals with RIF injection showed antibacterial effects than those without RIF injection, and comparing the biomodulation observed with CaSHA and CaSHA-VAN pellets, we are confident about the CaSHA-GEN pellets RIF biomodulation. Nevertheless, it would be interesting to have further studies where systemic RIF administration could be performed after a longer postoperative period allowing the complete elution of GEN from the CaSHA pellets.
Some limitations to the present study are noteworthy. We have tested only one HA-seeking antibiotics (RIF) in this study. However, future studies are planned to create a library of HA-seeking antibiotics and thus increase the availability of different classes of antibiotics for biomodulation. It needs to be acknowledged that this study does not provide in vivo data about the efficacy of biomodulation in infection prevention or treatment. However, being a proof-of-concept study, we believe our findings are valuable for future in vivo experiments. It should be noted that for the observed differences in RIF affinity between the pure n-/m-HA particles and biphasic CaSHA, we could only speculate that protein passivation may have played a role, but it calls for a separate study. Further studies are needed to explore the local apatite accretion threshold (both the total amount and the duration) for different systemically administered antibiotics.
This proof-of-concept study provided valuable information on the systemic biomodulation of locally implanted HA carriers. It is important to highlight that our findings are obtained with a clinically mimicking dose of RIF. Before clinical application, relevant in vivo bone infection models should verify these findings. Future studies should also aim to identify more HA-seeking antibiotics to cover a broader bacterial spectrum.
Data are available upon request.
SS, JH, and YL led the investigation. SS and YL developed the methodology. SS, JH, and YL curated the data. SS conducted the formal analysis. SS and LL acquired the funding. SS wrote the original draft. JH, YL, MC, MT, DBR, and LL reviewed and edited the paper. MC, MT, and LL supervised and collected the resources and validated the findings with DBR. DBR conceptualized the paper with LL and also administered the project.
Yang Liu, Deepak Bushan Raina, Magnus Tägil, and Lars Lidgren, are stockholders in Moroxite AB, Sweden. Lars Lidgren is a board member of BoneSupport AB, Sweden, and OrthoCell, Australia. All other authors declare that they have no conflict of interest.
All animal experiments were conducted with prior consent from the Swedish Board of Agriculture (Jordbruksverket) following the protocol number 15288/2019. All personnel working with animals had received prior training and certification as per the FELASA guidelines. Animals were housed in pairs in ventilated and temperature-controlled rooms with 12 h day and night cycles and free access to food and water.
Publisher's note: Copernicus Publications remains neutral with regard to jurisdictional claims in published maps and institutional affiliations.
Felix Tandberg is acknowledged for all his help during the animal and microbiological experiments.
This work was supported by Landshövding Per Westlings Minnesfond (grant number RMh 2021-0038) and Stina och Gunnar Wibergs Fond för Ortopedisk Forskning (Toffelfonden) (grant number F2022/768).
This paper was edited by Parham Sendi and reviewed by two anonymous referees.
Alagboso, F. I., Mannala, G. K., Walter, N., Docheva, D., Brochhausen, C., Alt, V., and Rupp, M.: Rifampicin restores extracellular organic matrix formation and mineralization of osteoblasts after intracellular Staphylococcus aureus infection, Bone. Joint. Res., 11, 327–341, https://doi.org/10.1302/2046-3758.115.Bjr-2021-0395.R1, 2022.
Anagnostakos, K., Hitzler, P., Pape, D., Kohn, D., and Kelm, J.: Persistence of bacterial growth on antibiotic-loaded beads: is it actually a problem?, Acta. Orthop., 79, 302–307, https://doi.org/10.1080/17453670710015120, 2008.
Anagnostakos, K., Wilmes, P., Schmitt, E., and Kelm, J.: Elution of gentamicin and vancomycin from polymethylmethacrylate beads and hip spacers in vivo, Acta. Orthop., 80, 193–197, https://doi.org/10.3109/17453670902884700, 2009.
Buchholz, H. W. and Engelbrecht, H.: Depot effects of various antibiotics mixed with Palacos resins, Chirurg, 41, 511–515, 1970.
Chen, Y., Wu, X., Li, J., Jiang, Y., Xu, K., and Su, J.: Bone-targeted nanoparticle drug delivery system: an emerging strategy for bone-related disease, Front. Pharmacol., 13, 909408, https://doi.org/10.3389/fphar.2022.909408, 2022.
Depypere, M., Kuehl, R., Metsemakers, W. J., Senneville, E., McNally, M. A., Obremskey, W. T., Zimmerli, W., Atkins, B. L., and Trampuz, A.: Recommendations for systemic antimicrobial therapy in fracture-related infection: A consensus from an international expert group, J. Orthop. Trauma, 34, 30-41, https://doi.org/10.1097/bot.0000000000001626, 2020.
Freeman, C. D., Nicolau, D. P., Belliveau, P. P., and Nightingale, C. H.: Once-daily dosing of aminoglycosides: review and recommendations for clinical practice, J. Antimicrob. Chemother., 39, 677–686, https://doi.org/10.1093/jac/39.6.677, 1997.
Funk, G. A., Menuey, E. M., Ensminger, W. P., Kilway, K. V., and McIff, T. E.: Elution of rifampin and vancomycin from a weight-bearing silorane-based bone cement, Bone. Joint. Res., 10, 277–284, https://doi.org/10.1302/2046-3758.104.Bjr-2020-0430.R1, 2021.
Gimza, B. D. and Cassat, J. E.: Mechanisms of antibiotic failure during Staphylococcus aureus osteomyelitis, Front. Immunol., 12, 638085, https://doi.org/10.3389/fimmu.2021.638085, 2021.
Gordon, O., Lee, D. E., Liu, B., Langevin, B., Ordonez, A. A., Dikeman, D. A., Shafiq, B., Thompson, J. M., Sponseller, P. D., Flavahan, K., Lodge, M. A., Rowe, S. P., Dannals, R. F., Ruiz-Bedoya, C. A., Read, T. D., Peloquin, C. A., Archer, N. K., Miller, L. S., Davis, K. M., Gobburu, J. V. S., and Jain, S. K.: Dynamic PET-facilitated modeling and high-dose rifampin regimens for Staphylococcus aureus orthopedic implant-associated infections, Sci. Transl. Med., 13, eabl6851, https://doi.org/10.1126/scitranslmed.abl6851, 2021.
Hanssen, A. D. and Spangehl, M. J.: Practical applications of antibiotic-loaded bone cement for treatment of infected joint replacements, Clin. Orthop. Relat. Res., 427, 79–85, https://doi.org/10.1097/01.blo.0000143806.72379.7d, 2004.
Henry, S. L. and Galloway, K. P.: Local antibacterial therapy for the management of orthopaedic infections. Pharmacokinetic considerations, Clin. Pharmacokinet., 29, 36–45, https://doi.org/10.2165/00003088-199529010-00005, 1995.
Hossain, M. S., Mahmud, M., Sultana, S., Bin Mobarak, M., Islam, M. S., and Ahmed, S.: Coupled effect of particle size of the source materials and calcination temperature on the direct synthesis of hydroxyapatite, R. Soc. Open. Sci., 8, 210684, https://doi.org/10.1098/rsos.210684, 2021.
Iundusi, R., Gasbarra, E., D'Arienzo, M., Piccioli, A., and Tarantino, U.: Augmentation of tibial plateau fractures with an injectable bone substitute: CERAMENT™, Three year follow-up from a prospective study, BMC Musculoskelet. Di., 16, 115, https://doi.org/10.1186/s12891-015-0574-6, 2015.
Kaczmarczyk, J., Sowinski, P., Goch, M., and Katulska, K.: Complete twelve month bone remodeling with a bi-phasic injectable bone substitute in benign bone tumors: a prospective pilot study, BMC Musculoskelet. Di., 16, 369, https://doi.org/10.1186/s12891-015-0828-3, 2015.
Kanellakopoulou, K. and Giamarellos-Bourboulis, E. J.: Carrier systems for the local delivery of antibiotics in bone infections, Drugs, 59, 1223–1232, https://doi.org/10.2165/00003495-200059060-00003, 2000.
Kotrych, D., Korecki, S., Ziętek, P., Kruk, B., Kruk, A., Wechmann, M., Kamiński, A., Kotrych, K., and Bohatyrewicz, A.: Preliminary results of highly injectable bi-phasic Bbne substitute (CERAMENT) in the treatment of benign bone tumors and tumor-like lesions, Open. Med.-Warsaw, 13, 487–492, https://doi.org/10.1515/med-2018-0072, 2018.
Liu, Y., Raina, D. B., Sebastian, S., Nagesh, H., Isaksson, H., Engellau, J., Lidgren, L., and Tägil, M.: Sustained and controlled delivery of doxorubicin from an in-situ setting biphasic hydroxyapatite carrier for local treatment of a highly proliferative human osteosarcoma, Acta Biomater., 131, 555–571, https://doi.org/10.1016/j.actbio.2021.07.016, 2021.
McNally, M. A., Ferguson, J. Y., Lau, A. C., Diefenbeck, M., Scarborough, M., Ramsden, A. J., and Atkins, B. L.: Single-stage treatment of chronic osteomyelitis with a new absorbable, gentamicin-loaded, calcium sulphate/hydroxyapatite biocomposite: a prospective series of 100 cases, Bone Joint. J., 98-b, 1289–1296, https://doi.org/10.1302/0301-620x.98b9.38057, 2016.
McNally, M. A., Ferguson, J. Y., Scarborough, M., Ramsden, A., Stubbs, D. A., and Atkins, B. L.: Mid- to long-term results of single-stage surgery for patients with chronic osteomyelitis using a bioabsorbable gentamicin-loaded ceramic carrier, Bone Joint. J., 104-b, 1095–1100, https://doi.org/10.1302/0301-620x.104b9.Bjj-2022-0396.R1, 2022.
Metsemakers, W. J., Kuehl, R., Moriarty, T. F., Richards, R. G., Verhofstad, M. H. J., Borens, O., Kates, S., and Morgenstern, M.: Infection after fracture fixation: Current surgical and microbiological concepts, Injury, 49, 511–522, https://doi.org/10.1016/j.injury.2016.09.019, 2018.
Nair, A. B. and Jacob, S.: A simple practice guide for dose conversion between animals and human, J. Basic Clin. Pharm., 7, 27–31, https://doi.org/10.4103/0976-0105.177703, 2016.
Neut, D., van de Belt, H., Stokroos, I., van Horn, J. R., van der Mei, H. C., and Busscher, H. J.: Biomaterial-associated infection of gentamicin-loaded PMMA beads in orthopaedic revision surgery, J. Antimicrob. Chemother., 47, 885–891, https://doi.org/10.1093/jac/47.6.885, 2001.
Nilsson, M., Zheng, M. H., and Tägil, M.: The composite of hydroxyapatite and calcium sulphate: a review of preclinical evaluation and clinical applications, Expert Rev. Med. Devic., 10, 675–684, https://doi.org/10.1586/17434440.2013.827529, 2013.
Osmon, D. R., Berbari, E. F., Berendt, A. R., Lew, D., Zimmerli, W., Steckelberg, J. M., Rao, N., Hanssen, A., and Wilson, W. R.: Diagnosis and management of prosthetic joint infection: clinical practice guidelines by the Infectious Diseases Society of America, Clin. Infect. Dis., 56, e1–e25, https://doi.org/10.1093/cid/cis803, 2013.
Qayoom, I., Teotia, A. K., Panjla, A., Verma, S., and Kumar, A.: Local and sustained delivery of rifampicin from a bioactive ceramic carrier treats bone infection in Rat Tibia, ACS Infect. Dis., 6, 2938–2949, https://doi.org/10.1021/acsinfecdis.0c00369, 2020.
Raina, D. B., Liu, Y., Isaksson, H., Tägil, M., and Lidgren, L.: Synthetic hydroxyapatite: a recruiting platform for biologically active molecules, Acta Orthop., 91, 126–132, https://doi.org/10.1080/17453674.2019.1686865, 2020a.
Raina, D. B., Liu, Y., Jacobson, O. L. P., Tanner, K. E., Tägil, M., and Lidgren, L.: Bone mineral as a drug-seeking moiety and a waste dump, Bone. Joint. Res., 9, 709–718, https://doi.org/10.1302/2046-3758.910.Bjr-2020-0097.R1, 2020b.
Sebastian, S., Malhotra, R., Dhawan, B., Sreenivas, V., Kapil, A., and Chaudhry, R.: Sonication of antibiotic loaded cement spacers: A valuable technique for detection of infection persistence in two-stage revision for infected joint arthroplasty, Indian J. Med. Microbi., 37, 99–101, https://doi.org/10.4103/ijmm.IJMM_18_313, 2019.
Sebastian, S., Sezgin, E. A., Stučinskas, J., Tarasevičius, Š., Liu, Y., Raina, D. B., Tägil, M., Lidgren, L., and W-Dahl, A.: Different microbial and resistance patterns in primary total knee arthroplasty infections – a report on 283 patients from Lithuania and Sweden, BMC Musculoskelet. Di., 22, 800, https://doi.org/10.1186/s12891-021-04689-5, 2021.
Sebastian, S., Tandberg, F., Liu, Y., Tägil, M., Lidgren, L., and Raina, D. B.: A Targeted drug delivery approach in deep bone infections using synthetic hydroxyapatite as a recruiting moiety, ORS 2022 Annual Meeting, 4–8 February, Tampa, Florida, Abstract no. 0031, 2022a.
Sebastian, S., Tandberg, F., Liu, Y., Raina, D. B., Tägil, M., Collin, M., and Lidgren, L.: Extended local release and improved bacterial eradication by adding rifampicin to a biphasic ceramic carrier containing gentamicin or vancomycin, Bone. Joint. Res., 11, 787–802, https://doi.org/10.1302/2046-3758.1111.BJR-2022-0101.R1, 2022b.
Sendi, P. and Zimmerli, W.: The use of rifampin in staphylococcal orthopaedic-device-related infections, Clin. Microbiol. Infect., 23, 349–350, https://doi.org/10.1016/j.cmi.2016.10.002, 2017.
Stravinskas, M., Horstmann, P., Ferguson, J., Hettwer, W., Nilsson, M., Tarasevicius, S., Petersen, M. M., McNally, M. A., and Lidgren, L.: Pharmacokinetics of gentamicin eluted from a regenerating bone graft substitute: In vitro and clinical release studies, Bone. Joint. Res., 5, 427–435, https://doi.org/10.1302/2046-3758.59.Bjr-2016-0108.R1, 2016.
Stravinskas, M., Tarasevicius, S., Laukaitis, S., Nilsson, M., Raina, D. B., and Lidgren, L.: A ceramic bone substitute containing gentamicin gives good outcome in trochanteric hip fractures treated with dynamic hip screw and in revision of total hip arthroplasty: a case series, BMC Musculoskelet. Di., 19, 438, https://doi.org/10.1186/s12891-018-2360-8, 2018.
Stravinskas, M., Nilsson, M., Vitkauskiene, A., Tarasevicius, S., and Lidgren, L.: Vancomycin elution from a biphasic ceramic bone substitute, Bone Joint. Res., 8, 49–54, https://doi.org/10.1302/2046-3758.82.Bjr-2018-0174.R2, 2019.
Vandiver, J., Dean, D., Patel, N., Bonfield, W., and Ortiz, C.: Nanoscale variation in surface charge of synthetic hydroxyapatite detected by chemically and spatially specific high-resolution force spectroscopy, Biomaterials, 26, 271–283, https://doi.org/10.1016/j.biomaterials.2004.02.053, 2005.
Zimmerli, W. and Sendi, P.: Role of Rifampin against staphylococcal biofilm Infections in vitro, in animal models, and in orthopedic-device-related infections, Antimicrob. Agents Ch., 63, e01746-18, https://doi.org/10.1128/aac.01746-18, 2019.