the Creative Commons Attribution 4.0 License.
the Creative Commons Attribution 4.0 License.
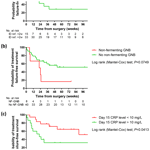
Osteocutaneous-flap-related osteomyelitis following mandibular reconstruction: a cohort study of an emerging and complex bone infection
Clément Javaux
Clémentine Daveau
Clotilde Bettinger
Mathieu Daurade
Céline Dupieux-Chabert
Fabien Craighero
Carine Fuchsmann
Philippe Céruse
Arnaud Gleizal
Nicolas Sigaux
Tristan Ferry
Florent Valour
Osteocutaneous flap (OCF) mandible reconstruction is at high risk for surgical site infection. This study aimed to describe diagnosis, management, and outcome of OCF-related osteomyelitis. All patients managed at our institution for an OCF-related osteomyelitis following mandible reconstruction were included in a retrospective cohort study (2012–2019). Microbiology was described according to gold-standard surgical samples, considering all virulent pathogens, and potential contaminants if present on at least two samples. Determinants of treatment failure were assessed by logistic regression and Kaplan–Meier curve analysis. The 48 included patients (median age 60.5 (IQR, 52.4–66.6) years) benefited from OCF mandible reconstruction mostly for carcinoma (; 56.3 %) or osteoradionecrosis (; 25.0 %). OCF-related osteomyelitis was mostly early (≤3 months post-surgery; ; 89.6 %), presenting with local inflammation (; 59.6 %), nonunion (wound dehiscence) or sinus tract (; 59.6 %), and/or bone or device exposure (; 44.7 %). Main implicated pathogens were Enterobacteriaceae (; 61.0 %), streptococci (; 53.7 %), Staphylococcus aureus (; 24.4 %), enterococci (; 22.0 %), non-fermenting Gram-negative bacilli (; 19.5 %), and anaerobes (; 19.5 %). Thirty-nine patients (81.3 %) benefited from surgery, consisting of debridement with implant retention (DAIR) in (64.1 %) cases, associated with 93 (IQR, 64–128) days of antimicrobial therapy. After a follow-up of 18 (IQR, 11–31) months, (50.0 %) treatment failures were observed. Determinants of treatment outcomes were DAIR (OR, 3.333; 95 % CI, 1.020–10.898) and an early infectious disease specialist referral (OR, 0.236 if ≤2 weeks; 95 % CI, 0.062–0.933).
OCF-related osteomyelitis following mandibular reconstruction represents difficult-to-treat infections. Our results advocate for a multidisciplinary management, including an early infectious-disease-specialist referral to manage the antimicrobial therapy driven by complex microbiological documentation.
- Article
(676 KB) - Full-text XML
- BibTeX
- EndNote
Osteocutaneous flaps (OCF), mostly consisting of free fibular flaps, are increasingly used for mandibular reconstruction after head and neck cancer surgery but also in rarer indications such as benign tumors, mandibular chronic osteomyelitis, radionecrosis/chemonecrosis of the jaw, or posttraumatic reconstruction. This complex technique is associated with a high risk of perioperative complications, reaching 54 % in some studies (Eskander et al., 2018; Suh et al., 2004), and especially a 13 % to 41 % risk of surgical site infection (SSI) (Kamizono et al., 2014; Karakida et al., 2010; Makiguchi et al., 2019; Cannon et al., 2017). This high SSI rate is probably due to the clean-contaminated surgical field with intrabuccal exposure. Furthermore, flap reconstruction gathers many procedure-associated risk factors of SSI – including long surgery duration, blood loss, and use of tracheostomy (Lee et al., 2011; Lin et al., 2018; Mücke et al., 2012) – and permits us to treat more aggressively advanced tumors in a population highly exposed to SSI due to advanced age, frequent co-morbidities, tobacco consumption, and impaired nutritional status (Kamizono et al., 2014; Makiguchi et al., 2019; Lee et al., 2011; Khariwala et al., 2016; Shum et al., 2014). Along with radiotherapy or chemotherapy for cancer management (Bourget et al., 2011; Benatar et al., 2013; Halle et al., 2017), such conditions can lead to infection despite an appropriate antimicrobial prophylaxis (Durand et al., 2015; Haidar et al., 2018). Osteomyelitis following OCF mandible reconstruction is associated with high morbidity and risk of flap failure (Kamizono et al., 2014; Karakida et al., 2010; Makiguchi et al., 2019; Cannon et al., 2017). As described for other postoperative bone and joint infections (BJIs) (Grammatico-Guillon et al., 2012), it may require revision surgery, prolonged broad-spectrum antimicrobial therapy, and extended hospital stay, leading to impaired quality of life and considerable medical expenditure.
Very few studies described mandibular OCF-related osteomyelitis, and there are no specific guidelines for its management. Consequently, practices are highly heterogeneous, often driven by prosthetic joint infection recommendations (Kapadia et al., 2016; ICM, 2022). However, anatomical conditions and pathophysiological pathways of these infections are very different, which should result in specific diagnosis and management considerations.
In this study, we aimed to describe clinical and microbiological diagnostic features, management, and outcome of OCF-related osteomyelitis following mandibular reconstruction in a referral center for the management of complex BJI.
2.1 Study design and data collection
All adult patients with mandibular OCF-related osteomyelitis followed up in our reference center for the management of complex BJI between 1 September 2012 and 31 July 2019; they were included in a retrospective observational cohort study. Infections requiring less than 1 month of antimicrobial therapy were considered skin and soft tissue infections (SSTIs), so these cases were excluded.
For each patient, data were collected from medical records and biological software in an anonymous standardized case report form. Co-morbidities were summarized by the Charlson co-morbidity index and the American Society of Anesthesiologists (ASA) score.
2.2 Surgical procedure
The harvesting step was performed through an anterior or posterior approach, with or without pneumatic tourniquet, depending on the operator's habits, using 2.0 magnifying glasses. A dose of platelet aggregation inhibitor was administered to the patient just before flap ischemia. Perforators were located visually in the distal third of the leg at the beginning of the procedure, with non-routine help of an ultrasound Doppler. The skin paddle was positioned with the most suitable perforator (size, pulsatility) in its center. If possible, two perforators were included. The skin paddle could be set intraorally or extraorally depending on the defect to be reconstructed, without tension on the suture line. If both sides had to be reconstructed, we used the skin paddle for extraoral reconstruction and a muscle for the intraoral side (either the tibialis posterior or a part of the soleus). The donor vessels were the fibular artery which divided just below the bifurcation of the tibiofibular trunk and one of the fibular veins. Arterial anastomoses were performed in a termino-terminal fashion using separate stitches of 8-0 or 9-0 non-absorbable sutures (Ethilon™, Ethicon Inc., Scotland, 1915). Venous anastomoses are either performed in a termino-terminal fashion on one of the proximal branches of the thyro-linguo-facial trunk or in a termino-lateral fashion on the internal jugular vein in second intention, with separate stitches of non-absorbable thread. Regarding bone reconstruction, most of the patients included in the study benefited from preoperative digital planning, custom-made cutting guide, and patient-specific plates with load sharing osteosynthesis. After surgery, flaps were monitored clinically to detect early microvascular failure, using the skin palette as control (heat, color, skin recolouration time). Additionally, Doppler flowmetry monitoring was performed twice daily during the postoperative period, during the entire stay (20 d on average).
2.3 Definitions
In the absence of consensual definition of OCF-associated osteomyelitis, diagnosis was based upon clinical, biological, imaging evidence, and/or culture of microorganisms from deep surgical samples, according to the definition of surgical site infection of the US Centers for Disease Control and Prevention (CDC) (Mangram et al., 1999).
On the basis of prosthetic joint infection chronological definitions (Kapadia et al., 2016; ICM, 2022), the delay between inoculation and symptoms was defined as early (≤3 months), delayed (3–12 months), and late (>12 months) postoperative infections. Similarly, infection was classified as “acute” when the delay between inoculation and management was ≤4 weeks.
All intraoperative tissue samples were inoculated onto a Columbia sheep's blood agar plate (read at days 1 and 2), two PolyVitex chocolate agar plates (read at days 1 and 2 for one plate and days 7 and 10 for the other), two blood agar plates for anaerobic incubation (read at days 3 and 5 for one plate and at days 7 and 10 for the other), and into a Schaedler anaerobic liquid broth. The broth was systematically subcultured on day 10 onto chocolate and blood agar plates for anaerobic incubation for 5 d. Identification and antibiotic susceptibility testing were performed following standard laboratory procedures (VITEK 2 system or VITEK MALDI-TOF MS; disk diffusion methods or ATB ANA device; bioMérieux, Marcy l'Etoile, France). Microbiological documentation was described, considering all virulent pathogens (i.e., Staphylococcus aureus, streptococci, and Gram-negative bacilli (GNB)) yielded out of deep surgical samples, and low virulent pathogens and/or potential contaminants (i.e., coagulase-negative staphylococci (CoNS), Corynebacterium spp., and Cutibacterium spp.) if present on at least two deep surgical samples, which is considered the gold standard (Osmon et al., 2013).
Antimicrobial therapy was considered empirical in the absence of previous microbiological documentation. It was considered appropriate when all pathogens highlighted by gold-standard sample cultures were targeted by at least one molecule.
Treatment failure was defined as symptom persistence under treatment (i.e., worsening or recurrence of local inflammatory symptoms, nonunion, sinus tract, abscess, purulent discharge, and bone and/or device exposure), infection relapse after treatment disruption, infection-related requirement of additional surgical procedure, flap loss, and infection-related death.
2.4 Statistical analysis
Descriptive statistics were used to estimate the frequencies of the study variables, described as numbers (%) for dichotomous values and medians (interquartile range, IQR) for continuous values. For the percentage calculation of each variable, the number of missing values was excluded from the denominator. Nonparametric statistical methods were used to compare the study groups (chi-squared test, Fisher's exact test, or Mann–Whitney U test, as appropriate). Determinants of treatment failure were assessed using: (i) logistic regression analysis, expressed as odds ratios (OR) and their 95 % confidence intervals (95 % CI), and (ii) Kaplan–Meier curve analysis representing the probability of treatment-failure-free survival with time, compared between groups using the log-rank (Mantel–Cox) test. A p value of ≤0.05 was considered significant. All analyses were performed using SPSS software version 17.0 (SPSS, Chicago, IL, USA) and GraphPad Prism version 5.3 (GraphPad Software, San Diego, CA, USA).
Table 1Description of the included population and comparison of patients with favorable outcome or treatment failure.
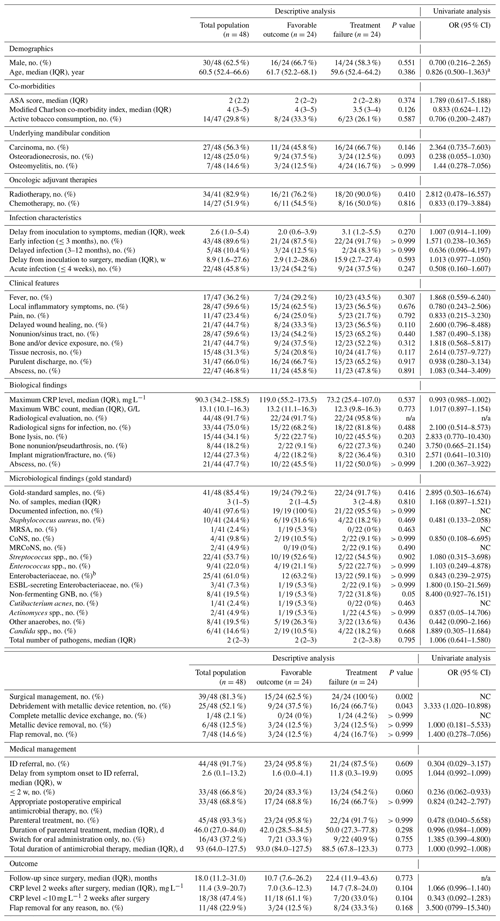
95 % CI, 95 % confidence interval; ASA, American Society of Anesthesiologists; CoNS, coagulase-negative staphylococci; CRP, C-reactive protein; EAT, empirical antimicrobial therapy; ESBL, extended-spectrum betalactamase; GNB, Gram-negative bacilli; ID, infectious disease; IQR, interquartile range; MRCoNS, methicillin-resistant coagulase-negative staphylococci; MRSA, methicillin-resistant Staphylococcus aureus; n/a, not applicable; NC, not calculable; OR, odds ratio; WBC, white blood cell. a Calculated for 10 additional years. b Including 7 Escherichia coli, 5 Proteus mirabilis, 5 Klebsiella pneumoniae, 4 Enterobacter cloacae, 2 Proteus vulgaris, 2 Citrobacter koseri, 2 Morganella morganii, 1 Halfnia alvei, 1 Citrobacter freundii, and 1 Klebsiella oxytoca.
3.1 Baseline patient characteristics
Forty-eight patients with mandibular OCF-associated osteomyelitis were included. Thirty of the 48 included patients (62.5 %) were male, with a median age of 60.5 (IQR, 52.4–66.6) years and a median modified Charlson co-morbidity index of 4 (IQR, 3–5).
Flap reconstruction was mostly performed for carcinoma (; 56.3 %), and 34 out of 41 patients (82.9 %) had previous neck irradiation. The major donor flap was fibula (; 95.8 %), with the two last patients having received clavi-pectoral and serrato-costal flaps. Cervical lymphadenectomy and tracheotomy were performed in (47.9 %) and (50.0 %) patients, respectively. Antimicrobial prophylaxis followed the French guidelines in 42 (97.7 %) of the 43 evaluable patients, mostly based on amoxicillin–clavulanate.
Patients and index surgery characteristics are summarized in Table 1.
3.2 Infection characteristics
OCF-related osteomyelitis was mostly early (; 89.6 %) and was considered acute in (45.8 %) cases. Main symptoms are presented in Table 1. Fever was present in (36.2 %) patients. The median maximum CRP level was 90.3 mg L−1 (IQR, 34.2–158.5). Clinical symptoms were associated with radiological signs for infection in 75.0 % of cases ().
Table 2Diagnostic values of superficial samples in comparison with deep surgical samples (gold standard).
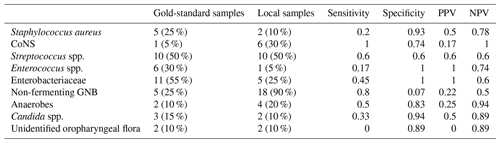
CoNS, coagulase-negative staphylococci; GNB, Gram-negative bacilli; NPV, negative predictive value; PPV, positive predictive value.
Gold-standard surgical samples were collected in (85.4 %) patients, with a median of three (IQR, 1–5) samples per patient, allowing for a microbiological documentation in (97.6 %) cases. Only three documented infections were monomicrobial. Main implicated pathogens were Enterobacteriaceae (; 61.0 %), streptococci (; 53.7 %) with a majority of milleri group (; 34.1 %), Staphylococcus aureus (; 24.4 %), Enterococcus faecalis (n=8/41; 19.5 %), anaerobes (; 19.5 %), and non-fermenting GNB (; 19.5 %). Only one patient had positive blood cultures (Actinomyces odontolyticus). Note that results of preoperative superficial microbiological samples were available in (54.2 %) patients. When comparing gold-standard and superficial sample culture results in the 20 (41.6 %) patients for whom both were available (Table 2), only four (20.0 %) were fully consistent. A 100 % predictive negative value was found for CoNS, only. Conversely, specificity and predictive positive values were good for Enterobacteriaceae and Enterococcus faecalis.
Histopathological analysis was performed in cases (50.0 %) with histological signs of infection in (54.5 %) cases including chronic inflammation in (36.4 %).
Out of the eight patients with no gold-standard microbiological documentation, all had local clinical and radiological signs of infection; four patients had histologically proven osteomyelitis, and six patients had local bacteriological samples highlighting invasive pathogens (milleri group streptococci, Enterobacteriaceae, and/or Pseudomonas aeruginosa).
3.3 Infection management
Thirty-nine (81.3 %) of the 48 included patients benefited from surgery, mostly consisting of debridement with implant retention (DAIR; ; 64.1 %). Flap had to be removed in 7/39 (17.9 %) cases during the initial septic surgery.
Empirical antimicrobial therapy was given for 16.5 (IQR, 7.8–31.8) days and considered appropriate in cases (68.8 %). The most commonly empirical regimen (; 45.8 %) was a combination of a broad-spectrum beta-lactam targeting non-fermenting GNB (piperacillin–tazobactam, cefepim, or carbapenem) with either vancomycin or daptomycin. Anaerobes were empirically targeted in (97.9 %) patients. Four (8.3 %) out of 48 patients empirically received an antifungal drug. After empirical antimicrobial therapy, the antimicrobial therapy was adapted to the definite microbiological documentation, with a complete oral administration possible in (37.2 %) patients, only. Total duration of antimicrobial therapy was 93 (IQR, 64.0–127.5) days. Antimicrobial therapy was driven by an infectious disease (ID) specialist in (91.7 %) cases, with a mean delay from symptoms to referral of 2.6 (IQR, 0.1–13.2) weeks. ID specialist advice was taken before surgery in (46.1 %) of the operated patients.
3.4 Outcome and determinants of treatment failure
In a median follow-up of 18 (IQR, 11.2–31.0) months, (50.0 %) treatment failures were observed, corresponding to symptom persistence (; 33.3 %), relapse (; 10.4 %), requirement for additional surgical procedure (; 42.6 %) including (8.3 %) flap removal, and (6.3 %) infection-related death.
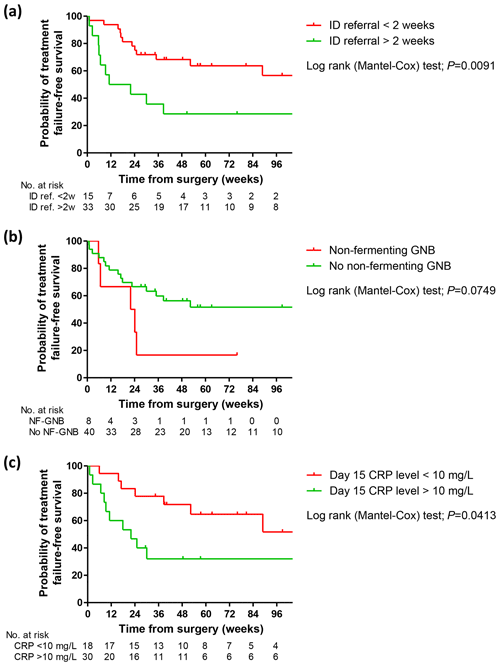
Figure 1Kaplan–Meier curves showing the probability of failure-free survival according to early ID specialist referral (a), non-fermenting GNB infection (b), and normalization of the biological inflammatory syndrome (c). ID, infectious disease; CRP, C-reactive protein; GNB, Gram-negative bacilli.
Baseline characteristics and infection diagnostic criteria were not different between the subsets of patients with or without treatment failure (Table 1). A higher proportion of patients treated with DAIR were observed in the treatment failure subset (; 66.7 % versus ; 37.5 %; p=0.043), which consequently appeared as a determinant of treatment failure (OR, 3.333; 95 % CI, 1.020–10.898, p=0.046). The only other determinant of treatment outcome was an early referral to ID specialist (OR, 0.236 if ≤2 weeks; 95 % CI, 0.062–0.933; p=0.035). Non-fermenting GNB infections tended to be associated with a higher risk of failure (OR, 8.400; 95 % CI, 0.927–76.151; p=0.058) (Table 1). Kaplan–Meier curve analysis confirmed these results and additionally suggested the non-negative state of the biological inflammatory syndrome after 2 weeks as another potential predictor of treatment failure (Fig. 1).
Note that the eight patients with no gold-standard microbiological diagnosis received 92 (IQR, 69.8–112.0) days of antimicrobials, after a surgery in four patients. Persistent infection was noted in three patients, including two requiring an additional surgery and one death.
Concerning the nine patients who did not benefit from surgery, all had early postoperative infection. They all received an appropriate antimicrobial therapy, based on the result of multiple local samples, available for all patients, and guided by an infectious disease specialist, for a total duration of 98 (IQR, 86–127) days. No treatment failure was observed after a follow-up of 15.3 (IQR, 6.2–26.0) months after the end of treatment. Two patients died of non-infectious causes. No specificity could be highlighted in this specific subset of patients.
OCF-related osteomyelitis represents an emerging BJI due to the increasing use of this surgical reconstruction technique, especially after head and neck cancer surgery. This infection can be considered complex, as it is mostly occurring in highly co-morbid patients and without consensual guidance for their difficult surgical management and broad-spectrum antimicrobial therapy to avoid treatment failure and flap loss.
We present here the first large series describing diagnosis, microbiological characteristics, management, and outcome of OCF-related osteomyelitis. Our results confirmed the poor prognosis of this infection, with a 50 % treatment failure rate despite complex medico-surgical support.
Diagnosis of OCF-related osteomyelitis is challenging and relies on an array of clinical, radiological, histological, and microbiological arguments. Clinical characteristics and infection chronology have to be known to avoid management delay. Even if the differential diagnosis with SSTI or early vascular complications can be difficult (Pohlenz et al., 2012), OCF-related osteomyelitis should be suspected in case of any incision incident including local inflammation, purulent discharge, nonunion or sinus tract, bone or device exposure, and abscess, as for other BJIs (Osmon et al., 2013). They mostly occur within 3 months post-surgery, consistent with previously published series, in which the superficial or deep nature of SSI and the existence of osteomyelitis were not stipulated (Durand et al., 2015; Lin et al., 2018). As expected for acute infections, bone abnormalities were not constant on imaging, with a third of patients presenting bone lysis only, but CT scan is also crucial to assess local complications (McCarty et al., 2019). Unfortunately, histopathological analysis was not available for all patients, impeding precise conclusions, but it might play an important role in doubtful cases as for other BJIs (Kapadia et al., 2016; Osmon et al., 2013; ICM, 2022).
Concerning microbiological documentation, the stringent definition used aimed to limit consideration of potential contaminants. Consistently with previously published series (Durand et al., 2015; Becker et al., 1978; Park et al., 2015), we highlighted a majority of polymicrobial infections, explained by intra-buccal exposure. As reported by Durand et al. (2015), a majority of GNB were surprisingly noted, along with the more expected streptococci, staphylococci, and anaerobes. Another questionable finding was the high proportion of enterococci and non-fermenting GNB. These microbiological considerations raise several issues. First, surgical-site contamination by local commensal flora might lead to polymicrobial infections, among which distinguishing sample contamination with saprophytic species from invasive isolates makes the microbiological diagnosis challenging The use of the IDSA (Infectious Diseases Society of America) guidelines for prosthetic joint infection diagnosis to interpret the microbiological result in the particular setting of head and neck surgery might not be appropriate (Osmon et al., 2013). Of note, as for other BJIs, is that sensitivity, specificity, and predictive values of superficial samples were insufficient to recommend them in the diagnostic strategy. Very few other studies analyzed the correlation of preoperative oral swabs and surgical deep samples in head and neck cancer SSI, and they did not demonstrate a good concordance either (Becker et al., 1978; Yang et al., 2013). Second, the high level of polymicrobism complicates the laboratory diagnostic process, leading to greater potential contaminants under the label “unidentified oropharyngeal flora”, which might underestimate the prevalence of some pathogens such as anaerobes, including Actinomyces spp. Third, the recommended antimicrobial prophylaxis based on amoxicillin–clavulanate might not be appropriate, and broader-spectrum molecules should be considered. Finally, this microbiological description advocates for the use of broad-spectrum empirical antimicrobial therapy awaiting definitive bacteriological diagnosis. A combination of piperacillin–tazobactam and daptomycin or cefepim with vancomycin and metronidazole could be adequate options to target GNB, streptococci, staphylococci, and anaerobes. Given the severity of the infection, this complex microbiological documentation, and its poor bone penetration, simple oral empirical antimicrobial therapy such as amoxicillin–clavulanate cannot be recommended.
OCF-related osteomyelitis was associated with a treatment failure rate of 50 %, and it was 22.9 % for flap loss. None of the baseline characteristics of patients and infection were found to influence clinical outcome in our series. Among these factors, prior radiotherapy could increase the risk of flap failure such as inducing fibrosis, damaging the microvasculature, or being associated with wound healing disorders (Schultze-Mosgau et al., 2002; Mueller and Schultze-Mosgau, 2009). However, if it represents a debated risk factor of SSI (Mücke et al., 2012; Bourget et al., 2011; Benatar et al., 2013; Halle et al., 2017), it was not associated with an increased risk of infection treatment failure. The impact of surgical strategy on treatment outcome has been poorly evaluated. In our series, DAIR had been highlighted with an increased risk of failure. However, the choice of surgical strategy in OCF-related osteomyelitis is challenged by the risk of vascular damage and flap loss in the early postoperative period. Unlike other device-associated infections, the benefit of complete foreign body removal has never been demonstrated and might not be required in most situations of early infections, except in the event of infection-induced flap loss. Similarly, antibiotic therapy features did not influence treatment outcome in our series. The appropriate total duration of antimicrobial therapy is unknown. Most included patients received a 3-month course of treatment, as recommended for other device-associated BJIs. Additionally, an early infectious disease specialist referral positively influences the outcome. This point highlights the importance of a multidisciplinary infection management with ear, nose, and throat surgeons and maxillofacial surgeons; microbiologists; radiologists; and ID specialists to help with infection diagnosis, determining optimal medico-surgical strategy, and limiting treatment failure. Such a dedicated trained team is required to personalize disease management, as highlighted for other complex BJI management by the dedicated French healthcare network (Ferry et al., 2019).
Some limitations of our study should be addressed. First, it includes the classical biases of retrospective and single-center design studies. Indeed, the long recruitment period and changes in practices and actors could make this series heterogeneous and our sample is relatively small. However, our series represents the first large description of this emerging complex infection and provides important insights into its management. Therefore, future investigations are needed to more precisely analyze the impact of different surgical strategies and medical management, even if larger sample size and prospective studies might be difficult to perform in this field. Second, the definition used for patient inclusion can be debated, as no consensual definition of OCF-associated osteomyelitis exists. We used a practical clinical point of view, with a mix of clinical, radiological, microbiological, and histological arguments, as none of the CDC surgical-site infection or other bone and joint infection definitions can be perfectly applied to this very specific entity. Finally, it was not possible to assess the main mechanism implicated in the infection occurrence, especially to differentiate direct inoculation from a consequence of microvascular issues of the flap, but both usually result in a severe postoperative infection. Patients with obvious early vascular failure were not included. Note that, in our center, rates of early failure (irreversible arterial or venous thrombosis ≤14 d), revision rate for anastomoses, and partial failure (i.e., loss of the skin paddle without the bony part of the flap) have been estimated at 6 %, 8 %, and 10 %, respectively.
Osteomyelitis following mandibular reconstruction with OCF represents difficult-to-treat infections with a high risk of treatment failure. Our results advocate for a multidisciplinary management, including an early ID specialist referral to manage the antimicrobial therapy driven by complex microbiological documentation, which might include a broad-spectrum empirical antimicrobial therapy targeting Gram-positive and Gram-negative bacteria, and anaerobes.
This study was subject to a declaration to the local Commission for Data Protection and Liberties (number 19-273) and is registered on https://clinicaltrials.gov (last access: 4 May 2022) (number NCT04373278). All patients received written information about the study. According to French legislation on non-interventional retrospective studies, no written informed consent was required for inclusion.
Underlying research data can be accessed on request from the corresponding author.
Lyon BJI study group: Coordinator: Tristan Ferry; Infectious Disease Specialists: Tristan Ferry, Florent Valour, Thomas Perpoint, Florence Ader, Sandrine Roux, Claire Triffault-Fillit, Agathe Becker, Anne Conrad, Cécile Pouderoux, Pierre Chauvelot, Paul Chabert, Johanna Lippman, Evelyne Braun, Marie Simon, Christian Chidiac; Surgeons: Clémentine Daveau, Philippe Céruse, Carine Fuchsmann, Thais Mouton, Jacques Blanc, Arnaud Gleizal, Mathieu Daurade, Jérôme Bourlet, Pierre Breton, Antoine Pierrefeu, Nicolas Sigaux, Sébastien Lustig, Elvire Servien, Cécile Batailler, Stanislas Gunst, Axel Schmidt, Matthieu Malatray, Eliott Sappey-Marinier, Michel-Henry Fessy, Anthony Viste, Jean-Luc Besse, Philippe Chaudier, Lucie Louboutin, Quentin Ode, Adrien Van Haecke, Marcelle Mercier, Vincent Belgaid, Arnaud Walch, Sébastien Martres, Franck Trouillet, Cédric Barrey, Ali Mojallal, Sophie Brosset, Camille Hanriat, Hélène Person; Microbiologists: Frédéric Laurent, Céline Dupieux-Chabert, Laetitia Béraud, Camille Kolenda, Jérôme Josse, Tiphaine Roussel-Gaillard; Anesthesiologists: Caroline Pic, Faustine Bredeche, Christian Bauer, Clotilde Bettinger, Mikhail Dziadzko, Caroline Macabéo; Radiologists: Fabien Craigheiro, Loic Boussel, Jean-Baptiste Pialat; Nuclear Medicine: Isabelle Morelec, Marc Janier, Francesco Giammarile; PK/PD specialists: Michel Tod, Marie-Claude Gagnieu, Sylvain Goutelle; Clinical Research Assistant: Eugénie Mabrut.
Acquisition, analysis, or interpretation of the data were conducted by CJ, CD, and CB, assisted by MD, CF, PC, AG, and NS for surgical data; FV and TF for infectiological data; FC for radiological data; and CDC for microbiological data.
The contact author has declared that neither they nor their co-authors have any competing interests.
Publisher's note: Copernicus Publications remains neutral with regard to jurisdictional claims in published maps and institutional affiliations.
This paper was edited by Parham Sendi and reviewed by two anonymous referees.
International Consensus Meeting (ICM): Second International Consensus Meeting on Musculoskeletal Infection, Part II: Hip and knee, https://icmphilly.com/hip-knee/, last access: 27 May 2022.
Becker, G. D., Parell, J., Busch, D. F., Finegold, S. M., and Acquarelli, M. J.: Anaerobic and aerobic bacteriology in head and neck cancer surgery, Arch. Otolaryngol., 104, 591–594, https://doi.org/10.1001/archotol.1978.00790100045010, 1978.
Benatar, M. J., Dassonville, O., Chamorey, E., Poissonnet, G., Ettaiche, M., Pierre, C. S., Benezery, K., Hechema, R., Demard, F., Santini, J., and Bozec, A.: Impact of preoperative radiotherapy on head and neck free flap reconstruction: a report on 429 cases, J. Plast. Reconstr. Aes., 66, 478–482, https://doi.org/10.1016/j.bjps.2012.12.019, 2013.
Bourget, A., Chang, J. T. C., Wu, D. B.-S., Chang, C. J., and Wei, F. C.: Free flap reconstruction in the head and neck region following radiotherapy: a cohort study identifying negative outcome predictors, Plast. Reconstr. Surg., 127, 1901–1908, https://doi.org/10.1097/PRS.0b013e31820cf216, 2011.
Cannon, R. B., Houlton, J. J., Mendez, E., and Futran, N. D.: Methods to reduce postoperative surgical site infections after head and neck oncology surgery, Lancet Oncol., 18, e405–e413, https://doi.org/10.1016/S1470-2045(17)30375-3, 2017.
Durand, M. L., Yarlagadda, B. B., Rich, D. L., Lin, D. T., Emerick, K. S., Rocco, J. W., and Deschler, D. G.: The time course and microbiology of surgical site infections after head and neck free flap surgery, Laryngoscope, 125, 1084–1089, https://doi.org/10.1002/lary.25038, 2015.
Eskander, A., Kang, S., Tweel, B., Sitapara, J., Old, M., Ozer, E., Agrawal, A., Carrau, R., Rocco, J. W., and Teknos, T. N.: Predictors of Complications in Patients Receiving Head and Neck Free Flap Reconstructive Procedures, Otolaryng. Head. Neck., 158, 839–847, https://doi.org/10.1177/0194599818757949, 2018.
Ferry, T., Seng, P., Mainard, D., Jenny, J.-Y., Laurent, F., Senneville, E., Grare, M., Jolivet-Gougeon, A., Bernard, L., Marmor, S., and CRIOAc network: The CRIOAc healthcare network in France: A nationwide Health Ministry program to improve the management of bone and joint infection, Orthop. Traumatol.-Sur., 105, 185–190, https://doi.org/10.1016/j.otsr.2018.09.016, 2019.
Grammatico-Guillon, L., Baron, S., Gettner, S., Lecuyer, A.-I., Gaborit, C., Rosset, P., Rusch, E., and Bernard, L.: Bone and joint infections in hospitalized patients in France, 2008: clinical and economic outcomes, J. Hosp. Infect., 82, 40–48, https://doi.org/10.1016/j.jhin.2012.04.025, 2012.
Haidar, Y. M., Tripathi, P. B., Tjoa, T., Walia, S., Zhang, L., Chen, Y., Nguyen, D. V., Mahboubi, H., Armstrong, W. B., and Goddard, J. A.: Antibiotic prophylaxis in clean-contaminated head and neck cases with microvascular free flap reconstruction: A systematic review and meta-analysis, Head Neck., 40, 417–427, https://doi.org/10.1002/hed.24988, 2018.
Halle, M., Eriksson, B. O., Docherty Skogh, A.-C., Sommar, P., Hammarstedt, L., and Gahm, C.: Improved Head and Neck Free Flap Outcome–Effects of a Treatment Protocol Adjustment from Pre- to Postoperative Radiotherapy, Plast. Reconstr. Surg. Glob. Open, 5, e1253, https://doi.org/10.1097/GOX.0000000000001253, 2017.
Kamizono, K., Sakuraba, M., Nagamatsu, S., Miyamoto, S., and Hayashi, R.: Statistical analysis of surgical site infection after head and neck reconstructive surgery, Ann. Surg. Oncol., 21, 1700–1705, https://doi.org/10.1245/s10434-014-3498-8, 2014.
Kapadia, B. H., Berg, R. A., Daley, J. A., Fritz, J., Bhave, A., and Mont, M. A.: Periprosthetic joint infection, Lancet, 387, 386–394, https://doi.org/10.1016/S0140-6736(14)61798-0, 2016.
Karakida, K., Aoki, T., Ota, Y., Yamazaki, H., Otsuru, M., Takahashi, M., Sakamoto, H., and Miyasaka, M.: Analysis of risk factors for surgical-site infections in 276 oral cancer surgeries with microvascular free-flap reconstructions at a single university hospital, J. Infect. Chemother., 16, 334–339, https://doi.org/10.1007/s10156-010-0108-y, 2010.
Khariwala, S. S., Le, B., Pierce, B. H. G., Isaksson Vogel, R., and Chipman, J. G.: Antibiotic Use after Free Tissue Reconstruction of Head and Neck Defects: Short Course vs. Long Course, Surg. Infect., 17, 100–105, https://doi.org/10.1089/sur.2015.131, 2016.
Lee, D. H., Kim, S. Y., Nam, S. Y., Choi, S.-H., Choi, J. W., and Roh, J.-L.: Risk factors of surgical site infection in patients undergoing major oncological surgery for head and neck cancer, Oral Oncol., 47, 528–531, https://doi.org/10.1016/j.oraloncology.2011.04.002, 2011.
Lin, S.-C., Chang, T.-S., Yang, K.-C., Lin, Y.-S., and Lin, Y.-H.: Factors contributing to surgical site infection in patients with oral cancer undergoing microvascular free flap reconstruction, Eur. Arch. Otorhinolaryngol., 275, 2101–2108, https://doi.org/10.1007/s00405-018-5035-z, 2018.
Makiguchi, T., Yokoo, S., Kanno, Y., Kurihara, J., and Suzuki, K.: Risk Factors for Surgical Site Infection in Patients Undergoing Free and Pedicled Myocutaneous Flap Reconstruction After Oral Cancer Resection, J. Oral Maxil. Surg., 77, 1075–1081, https://doi.org/10.1016/j.joms.2018.12.028, 2019.
Mangram, A. J., Horan, T. C., Pearson, M. L., Silver, L. C., and Jarvis, W. R.: Guideline for Prevention of Surgical Site Infection, 1999, Centers for Disease Control and Prevention (CDC) Hospital Infection Control Practices Advisory Committee, Am. J. Infect. Control, 27, 97–132, 1999.
McCarty, J. L., Corey, A. S., El-Deiry, M. W., Baddour, H. M., Cavazuti, B. M., and Hudgins, P. A.: Imaging of Surgical Free Flaps in Head and Neck Reconstruction, Am. J. Neuroradiol., 40, 5–13, https://doi.org/10.3174/ajnr.A5776, 2019.
Mücke, T., Rau, A., Weitz, J., Ljubic, A., Rohleder, N., Wolff, K.-D., Mitchell, D. A., and Kesting, M. R.: Influence of irradiation and oncologic surgery on head and neck microsurgical reconstructions, Oral Oncol., 48, 367–371, https://doi.org/10.1016/j.oraloncology.2011.11.013, 2012.
Mueller, C. K. and Schultze-Mosgau, S.: Radiation-induced microenvironments–the molecular basis for free flap complications in the pre-irradiated field?, Radiother. Oncol., 93, 581–585, https://doi.org/10.1016/j.radonc.2009.08.009, 2009.
Osmon, D. R., Berbari, E. F., Berendt, A. R., Lew, D., Zimmerli, W., Steckelberg, J. M., Rao, N., Hanssen, A., and Wilson, W. R.: Diagnosis and Management of Prosthetic Joint Infection: Clinical Practice Guidelines by the Infectious Diseases Society of America, Clin. Infect. Dis., 56, e1–e25, https://doi.org/10.1093/cid/cis803, 2013.
Park, S. Y., Kim, M. S., Eom, J. S., Lee, J. S., and Rho, Y. S.: Risk factors and etiology of surgical site infection after radical neck dissection in patients with head and neck cancer, Korean J. Intern. Med., 31, 162–169, https://doi.org/10.3904/kjim.2016.31.1.162, 2015.
Pohlenz, P., Klatt, J., Schön, G., Blessmann, M., Li, L., and Schmelzle, R.: Microvascular free flaps in head and neck surgery: complications and outcome of 1000 flaps, Int. J. Oral. Maxil., 41, 739–743, https://doi.org/10.1016/j.ijom.2012.02.012, 2012.
Schultze-Mosgau, S., Grabenbauer, G. G., Radespiel-Tröger, M., Wiltfang, J., Ries, J., Neukam, F. W., and Rödel, F.: Vascularization in the transition area between free grafted soft tissues and pre-irradiated graft bed tissues following preoperative radiotherapy in the head and neck region, Head Neck., 24, 42–51, https://doi.org/10.1002/hed.10012, 2002.
Shum, J., Markiewicz, M. R., Park, E., Bui, T., Lubek, J., Bell, R. B., and Dierks, E. J.: Low prealbumin level is a risk factor for microvascular free flap failure, J. Oral. Maxil., 72, 169–177, https://doi.org/10.1016/j.joms.2013.05.022, 2014.
Suh, J. D., Sercarz, J. A., Abemayor, E., Calcaterra, T. C., Rawnsley, J. D., Alam, D., and Blackwell, K. E.: Analysis of outcome and complications in 400 cases of microvascular head and neck reconstruction, Arch. Otolaryngol., 130, 962–966, https://doi.org/10.1001/archotol.130.8.962, 2004.
Yang, C.-H., Chew, K.-Y., Solomkin, J. S., Lin, P.-Y., Chiang, Y.-C., and Kuo, Y.-R.: Surgical site infections among high-risk patients in clean-contaminated head and neck reconstructive surgery: concordance with preoperative oral flora, Ann. Plast. Surg., 71 Suppl 1, S55–60, https://doi.org/10.1097/SAP.0000000000000046, 2013.